Safety Based Limits for the Control of Impurities in Drug Substances and Drug Products: A Review-Juniper Publishers
JUNIPER PUBLISHERS-OPEN ACCESS JOURNAL OF PHARMACY &
PHARMACEUTICAL SCIENCES
Introduction
Paracelsus, the medieval physician, who is often
viewed as the father of modern toxicology, was the first person to
appreciate that "the dose makes the poison". This essentially means that
very toxic materials can be used therapeutically at very low
concentrations and conversely even safe materials can be toxic if
overdosed. This in turn led to Haber's law, which basically states that,
the incidence and/or severity of any toxic effect is dependent on the
total exposure to the toxic agent; that is, the exposure concentration
(c) rate(or dose) multiplied by the duration time (t) of exposure (i.e.
cxt). This law is often utilised in setting exposure limits for toxic
components. The major caveat, is that establishing acceptable daily
intakes (ADIs) for long-term exposures to a toxic substance when only
data from short-term studies are available, does require the use of an
uncertainty or safety factor.
For example, cancer risk estimates are typically
based on the average lifetime daily dose (LDD), which in turn is derived
from the total cumulative exposure, using Haber's law, i.e. cxt. Gaylor
[1]
proposed a modified Haber’s law to better extrapolate safe levels based
on shorter term exposure intervals and this takes the form of:
c3x t=c’3x t'
where c and t are the known safe exposure levels(c)
based on the longer exposure duration (t) and c’ and t' are the
projected safe concentration (c’) based on the pre-defined shorter
exposure duration (t’). Haber’s law is equally germane to impurities as
it is to medicinal products.
Impurities in New Drug Substances and New Drug Products (Ich Q3a/ Ich Q3b)
One of the first international guidance that used
safety based limits for impurities was the international conference on
harmonization (ICH) Q3A [2].
This provided an overview of the typical impurities that were found in
new drug substances and their controls. Impurities were evaluated based
on both chemistry considerations, including "classification and
identification of impurities, report generation, listing of impurities
in specifications, and a brief discussion of analytical procedures";
and safety considerations, including "specific guidance for qualifying
those impurities that were not present, or were present at substantially
lower levels, in batches of a new drug substance used in safety and
clinical studies"
Impurities were further delineated into identified
and unidentified classes, both of which were included as specification
tests [3].
This includes unidentified impurities that were known to be present at
levels greater than pre-defined reporting, identification and
qualification threshold (Table 1).
Those unidentified impurities are often defined on the drug substance
specification "by an appropriate qualitative analytical descriptive
label (e.g., "unidentified A", "unidentified with relative retention of
0.9")"
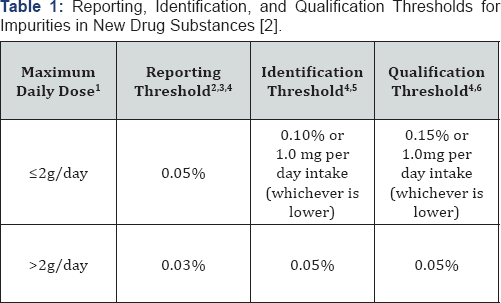
1. The amount of drug substance administered per day;
2. Reporting Threshold: A limit above (>) which an
impurity should be reported. Reporting threshold is the same as
reporting level in Q2B (4);
3. Higher reporting thresholds should be scientifically justified;
4. Lower thresholds can be appropriate if the impurity is unusually toxic;
5 Identification Thresholds: A limit above (>) which an impurity should be identified;
6 Qualification Thresholds: A limit above (>) which an impurity should be qualified
The reporting threshold was linked to the capability of the supporting analytical methodology [4].
Identification threshold was the limit where the unknown impurity was
required to be identified by appropriate analytical methodology.
Whereas, the qualification threshold necessitated acquiring and
evaluating pre-clinical safety data that "establishes the biological
safety of an individual impurity or a given impurity profile at the
level(s) specified".
Interestingly, although the derivation of the
reporting threshold was linked to method capability, the derivation of
the identification and qualification threshold limits were never fully
delineated, apart from linkage with the maximum daily dose of the
product. In addition, for those impurities "known to be unusually potent
or to produce toxic or unexpected pharmacological effects, the
quantitation/detection limit of the analytical procedures should be
commensurate with the level at which the impurities should be
controlled". Again, the implicit meaning of this statement was never
fully articulated, but it was the genesis for the subsequent guidance on
mutagenic impurities, initially termed genotoxic impurities [5].
Similar guidance was provided for impurities
typically found in new drug products. These impurities are usually
termed degradation products [6]. There was greater delineation of the thresholds in terms of dose (Table 2).
However, it was never fully explained why the various thresholds, in
terms of maximum daily dose, could not be aligned. Thus there is the
confusing scenario that the reporting thresholds are above or below 1g;
whereas, the identification thresholds are divided into four (>2g,
>10mg- 2g, 1mg-10mg and <1mg); whilst the qualification thresholds
were also divided into four, but were not aligned with the classes
defined in the identification thresholds (>2g, >100mg-2g, 10mg-
100mg and <10mg).

1 The amount of drug substance administered per day;
2 Thresholds for degradation products are expressed
either as a percentage of the drug substance or as total daily intake
(TDI) of the degradation product. Lower thresholds can be appropriate if
the degradation product is unusually toxic;
3 Higher thresholds should be scientifically justified.
The other confusing aspect was that the maximum daily
dose (mg/day) and the maximum strength of a product (mg) are often not
the same value. Thus for instance, the anti-malarial drug quinine
sulfate [7]
has a maximum therapeutic dose from the product label of 648mg every
8hours, i.e. 1944mg/day; whereas, the highest dose strength are
324mg/capsule. The analysts testing and releasing quinine sulfate
capsules will do so on the commercial product (324mg/capsule), not the
maximum dose taken by the patient (1944mg/day). In addition, although
reporting thresholds are always measured as percentage values and are
easily aligned with the data output from the method used by the analyst;
identification and qualification thresholds are measured in either
percentage values or mg/day values (Table 3).
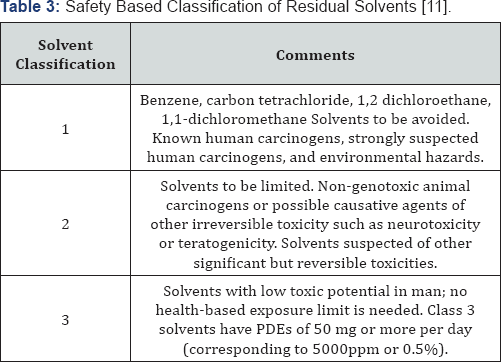
The other aspect of having safety based limits for
impurities is that it does not reflect the duration of treatment use for
that drug and results in the same limits being proposed irrespective of
whether the drug is proscribed pro ne rata (PRN) or as required,
e.g. for constipation, mild pain, etc., or through life time
treatments, e.g. for treatment of high blood pressure, etc. ICH Q3A [2] and Q3B [6]
were always intended to be only applicable to marketed products, but
the regulatory expectations during clinical development often exceed
what is actually required. For example, it isn't unusual to see the
following expectations [8]: "For phase I expect structure (or identifier) and
origin For phase II expect Limit of Detection and Quantification and
actual impurity levels to be established (aligned with ICH Q3A, Q3B,
etc)".
However, by phase II the final synthetic route and
process of the drug substance are rarely identified or optimised, and
the attrition rate of phase II drugs is still very high [9]. What the regulatory guidance enshrined in ICH M3 [10],
actually states with respect to impurities is, "If specific studies are
warranted to qualify an impurity or degradant, generally these studies
are not warranted before phase 3 unless there are changes that result in
a significant new impurity profile (e.g., a new synthetic pathway, a
new degradant formed by interactions between the components of the
formulation). In these latter cases, appropriate qualification studies
can be warranted to support phase 2 or later stages of development"
Residual Solvent Impurities (ICH Q3C)
Although residual solvents are mentioned in ICH Q3A [2], a separate guideline, ICH Q3C [11],
was developed to provide safety based guidance on the allowable limits
of common residual solvents within pharmaceuticals. As there are "no
therapeutic benefits from residual solvents, all residual solvents
should be removed to the extent possible to meet product specifications,
good manufacturing practices, or other quality based requirements".
Additionally, ICH Q3C recommends the use of less toxic solvents. Thus,
solvents that are known to be highly toxic (Class 1) should be avoided
during the production of drug substances, excipients, and especially
drug products, unless their usage can be justified using a risk-benefit
assessment [12].
In addition, some solvents with intermediate toxicity (Class 2) should
also be limited from a patient safety perspective. Ideally, the least
toxic solvents (Class 3) should always be used where practical.
Recommended limits for all solvents may change as additional safety data
become available. In addition, supporting safety data for new solvents
may be added to the guidance.
Although tolerable daily intake (TDI) and acceptable
daily intake (ADI) were both in common usage, ICH Q3C (12) introduced a
new term, permitted daily exposure (PDE) to avoid confusion of differing
values for ADI's for the same substance. In addition to avoidance of
class 1 solvents, the concept of "as low as reasonably practicable"
(ALARP) was introduced and is applied to class 2 solvents and often to
class 3 solvents. Indeed, regulatory agencies will often use process
capability arguments to drive down residual solvent levels below the
safety based limits [13] derived from ICH Q3C.
Residual Elemental Impurities (ICH Q3D)
Residual elemental impurities were also mentioned in ICH Q3A [2],
but again a separate guideline, ICH Q3D (14) was developed to provide
safety based guidance on the allowable limits of residual elements
within pharmaceuticals. As elemental impurities provide no therapeutic
benefit to the patient, "their levels in the drug product should be
controlled within acceptable limits" [14].
The ICH Q3D guideline is sub-divided into three
parts: the derivation and assessment of toxicity data; the establishment
of a PDE for each elemental impurity derived for three different routes
of administration (oral, inhaled and parenteral); and application of a
risk based approach to control elemental impurities (as per ICH Q9
(12)). One difference from ICH Q3C [11]
is that applicants are not expected to tighten the safety based limits
based on process capability considerations, as long as the residual
elemental impurities do not exceed the PDE values. However, in certain
cases, levels below the PDE may be warranted when lower levels have been
shown to positively impact on other critical quality attributes (CQAs)
of the drug product; for example, element catalyzed drug degradation
(this is particularly common with oxidative degradation mechanisms [15].
In addition, for those elements with higher PDEs, lower limits may have
to be assessed from a pharmaceutical quality perspective. Residual
elements are classified into 5 different categories: class 1, 2a, 2b, 3
and others (Table 4).
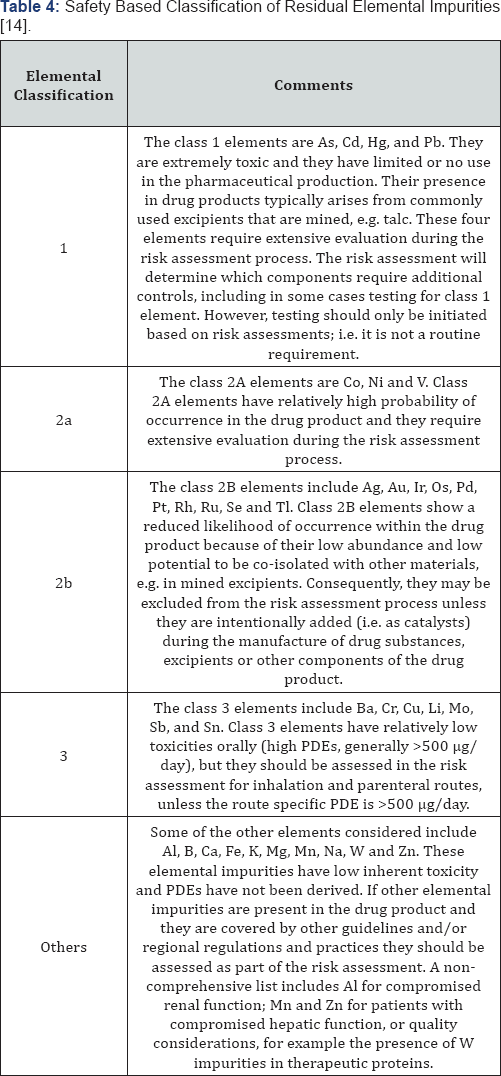
ICH Q3D [14]
provides a platform for developing an ICH Q9 (12) aligned risk-based
control strategy to limit elemental impurities within the drug product.
Although, the guidance had highlighted the risk inherent from both drug
substance and excipients, the reality based on a multi-product
assessment is that the risk is low. Li et al. [16]
tested 190 samples from 31 different excipients and 15 samples from
eight different drug substances for residual elemental impurities. The
results show relatively low levels of elemental impurities are present
in the samples tested.
Residual mutagenic impurities (ICH M7)
ICH M7 [5]
is focused on DNA reactive impurities that can potentially cause DNA
damage, when present at low levels, and thus can potentially cause
cancer in man. Importantly, other types of toxic impurities that are
non-mutagenic will typically have a threshold mechanism and as such
usually do not pose carcinogenic risk in man, at the levels typically
seen for impurities.
A Threshold of Toxicological Concern (TTC) approach
was introduced to describe an "acceptable intake for any unstudied
chemical that poses a negligible risk of carcinogenicity or other toxic
effects", this equates to a virtually safe dose (VSD). The methodologies
that underpin the TTC are universally considered to be very
conservative, as they use a simple linear extrapolation from the TD50 dose (i.e. dose giving a 50% tumor incidence) to a 1 in 106 likelihood of cancer occurrence.
A default TTC value of 1.5μg/day corresponding to a theoretical 10-5
excess lifetime risk of cancer can therefore be justified for mutagenic
impurities. Some high potency groups referred to as the "cohort of
concern", e.g. aflatoxin-like-, N-nitroso-, and alkyl-azoxy compounds;
were identified where the default TTC would still pose a significant
carcinogenic risk. These high potency compounds were excluded from the
TTC approach.
ICH M7 [5] bases acceptable intakes for mutagenic impurities on established risk assessment approaches (see ICH Q9 [12].
As such, acceptable risk during the early development phase is
established at a higher theoretically calculated risk level of
approximately one additional cancer incidence per million, i.e. 1 in 106
risk levels. For later stages in development (Phase III) and for
commercial products, the risk level is reduced to one in one hundred
thousand, i.e. 1 in 105 risk levels. It is worth
highlighting, that these risk levels represent a small theoretical
increase in risk when compared to the overall lifetime incidence of
developing cancer in man, which is greater than 1 in 3.
The initial risk assessment is undertaken on the drug
substance synthetic pathway to identify real or potential impurities
that may be reactive and thereby mutagenic in nature. In parallel, the
formulation and manufacturing process are also assessed for the
formation of any reactive degradants (both real and potential), that
could be realistically expected to form during long term, real-time
storage conditions. In silico structure- based assessments, i.e. Derek
Nexus, Sarah Nexus, etc., are used for predicting mutagenicity based
upon QSAR (quantitative structure activity relationships) approaches.
These findings are then reviewed by toxicology experts to provide any
additional understanding as to the relevance of these predictions (both
positive and negative), and in the case of contradictory outcomes to
understand those differences. Based on this assessment, impurities are
categorised into five different classes in order of decreasing
regulatory concern (Table 5).
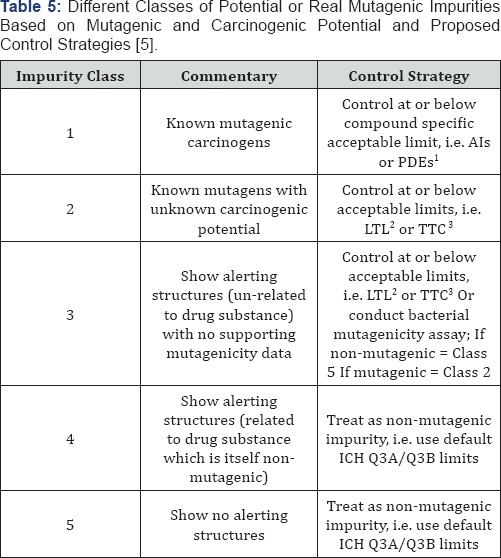
1. For class 1 compounds, i.e. those which are known
mutagenic carcinogens, an AI (acceptable intake) or a PDE (permitted
daily exposure)approach has been introduced (ICH M7(R1) (17)). These
limits are based on either (i) linear extrapolations from TD50 (AI) or
(ii) threshold-based PDEs. There are 10 compounds covered by the AI
approach and a further 3 covered by the PDE approach.
2. LTL (less than lifetime limits)
3. TTC (Threshold of Toxicological Concern)
It is anticipated that monitoring and control
strategies (including analytical methods) will be less developed during
earlier clinical phases, where overall development experience is of
necessity limited, compared to later clinical phases and commercial
manufacture. ICH M7 [17]
proposesa control strategy using four control options for mutagenic
impurities, of these only one includes control of the mutagenic impurity
on the API specification (option 1). Options 2 and 3 define some levels
of in-process control; whereas, option 4 is centred on process
understanding alone, typically termed "Purge Arguments" [18].
It is should be emphasised that these established
cancer risk assessments are based on lifetime exposures, i.e. 75 years.
Thus, Less-Than-Lifetime (LTL) exposure based limits can be derived both
during development and commercial use. LTLs can have higher acceptable
intakes of mutagenic impurities and still maintain comparable risk
levels, which is obviously an application of Haber's law. Therefore, the
carcinogenic effect is predicated on both duration of exposure and
dose. Thus for example, "if the compound specific acceptable intake is
15 |ig/day for lifetime exposure, the less than lifetime limits (Table 6)
can be increased to a daily intake of 100 |ig (>1-10 years treatment
duration), 200 |ig (>1-12 months) or 1200|ig (<1month)" [5].
This LTL approach may also be appropriate "in diseases with reduced
life expectancy, limited therapeutic alternatives or chronic diseases
with late onset" [19].
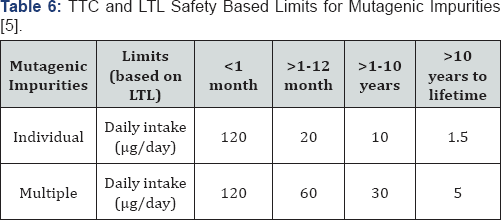
It is worth emphasising that exceeding the default
TTC or LTL limits is not necessarily linked with an increased cancer
risk in man, given the extremely conservative suppositions employed in
the evolution and derivation of the TTC or LTL values. For instance,
higher exposure to a potential mutagenic impurity, e.g. formaldehyde,
may be reasonable when exposure can be significantly greater from other
sources, e.g. endogenous metabolism, food, etc. The most likely increase
in cancer incidence is actually much less than 1 in 100,000. In
addition, in cases where a mutagenic compound is a non-carcinogen in a
rodent bioassay, there would be no predicted increase in cancer risk.
Based on all the above considerations, any exposure to an impurity that
is later identified as a mutagen is not necessarily associated with an
increased cancer risk for patients already exposed to the impurity. A
risk assessment would determine whether any further actions would be
taken
In principle, ICH M7 does not apply to advanced
cancer therapeutic indications (covered by ICH S9 (20)), where the drug
is itself genotoxic. ICH M7 does not apply to established excipients,
flavouring agents and certain biological products, including herbal
medicines. Existing commercial products are also exempted, apart from
where there are new safety data (including new mutagenic data) for
existing impurities; significantly, structural alerts alone do not
trigger regulatory concern. However, ICH M7 does cover changes to
marketed products, including new marketing applications and postapproval
submissions.
Impurities in oncology products (ICH S9)
ICH S9 [20]
was developed to provide guidance for nonclinical studies for the
development of anticancer pharmaceuticals used in clinical trials for
the treatment of patients with advanced disease and limited therapeutic
options. During the development of oncology products, supporting
clinical studies often involve cancer patients whose prognosis is poor
and projected lifetime is short (<2 years).
As such, the guideline objectives are to facilitate
and accelerate the development of these anticancer pharmaceuticals
whilst protecting patients from unnecessary adverse effects. In
addition, ethical use of animals, in accordance with the 3R principles
(reduce/refine/replace) are paramount. Importantly, the principles
described in other ICH guidelines need to be considered in the
development of oncology products; whereas, those specific situations
where requirements for nonclinical testing may diverge from other
guidance are described in ICH S9.
Additionally, the dose levels used in these clinical oncology studies are often at the top end of the tolerable range [21]
and often result in adverse effect dose levels. Hence, "the type,
timing and flexibility called for in the design of nonclinical studies
of anticancer pharmaceuticals can differ" significantly from nononcology
pharmaceuticals. Historically, limits for impurities (see ICH Q3A [2] and Q3B [6]
have been based on a negligible risk to the patient. In oncology
products this consideration, whilst important, is not as important as
patient wellbeing and exceeding the ICH Q3A [2]/Q3B [6] limits for impurities may be applicable and an appropriate justification should be included in the marketing application.
This explanation should include an overview of the
disease being treated, including patient prognosis, the nature of the
drug itself (pharmacology, genotoxicity and carcinogenicity, etc.), the
total duration of treatment, and the impact of any reduction in impurity
levels on manufacturability. Furthermore, the qualification of these
impurities may include reflections on the concentration tested in
supporting nonclinical studies compared to the levels seen in clinical
batches. In addition, TTC, LTL and AI/PDE limits for mutagenic
impurities (see ICH M7 [5])
are inappropriate for oncology products and justifications can be used
to set higher limits. Interestingly, the guidance does not specifically
say that the applicant can default to ICH Q3A [2]/ Q3B [6] limits, although this is often inferred. Impurities that are also metabolites can be considered to be suitably qualified.
Interestingly, regulators have been very unwilling to extend the philosophy of ICH S9 [20] beyond oncology products, for instance into rare diseases [22], where lifetime expectancy can be similarly short, i.e. <2 years and where patient expectations are equally high.
New Reflections on Impurities
Harvey et al. [23]
used a variety of chemical databases to demonstrate that the 1mg/day
impurity level for an unqualified impurity of unknown toxicity, proposed
by ICH Q3A [2] (Table 1)
is indeed a robust prediction of a virtually safe dose (VSD) for
non-mutagenic impurities. Then using the modified Haber's law, where C=1
mg and t=75 years (i.e., 27375 days) and t is 6 months or 182 days they
determined a VSD for this shorter exposure interval of 5 mg/day (i.e. 5
times higher than existing ICH Q3A limit). However, for very potent
drugs with effective doses of <1mg, a 5mg/day limit for a related
impurity isn't realistic from either a safety or quality perspective.
Therefore, the authors also introduced a percentage cut off based on 5x
the ICH Q3A qualification threshold of 0.15%; i.e. 0.7%. Thus the
proposed limits for drug substances are 5mg or 0.7%, whichever is lower.
This allows applicants to adopt the existing ICH Q3A
guideline which were developed for commercial products and apply them to
development products, in much the same way that the ICH M7 guidelines
allows LTL limits for mutagenic impurities, for early clinical
development. For drug products, similar LTL limits for non-mutagenic
impurities can be derived based on a modification of Haber’s law. The
additional constraint of a percentage limit of 0.7% need not be applied
to drug products as the more potent the drug substance becomes, the
lower the dose required. The authors therefore suggested a limit of 5 mg
or 2%, whichever is lower, for exposure intervals of <6 months, for
general drug substance impurities, i.e. non-mutagenic.
In addition to absolute amounts of unknown
impurities, the other key focus is those impurities with unusually high
and/ or specific toxicities. Whilst it is recognised that mutagenic
impurities constitute the greatest threat to patient safety and they
have been addressed via ICH M7; there are other classes of non-mutagenic
impurities that will still give cause for concern. The three principal
classes of toxic impurities are
(i) polyhalogenated, dibenzodioxins, dibenzofurans
and biphenyls that are non-mutagenic carcinogens, which have specific
regulatory framework with respect to acceptable exposure levels [24],
(ii) organophosphates or carbamates that are neurotoxins and have their own threshold of concern [25]
and (iii) β- lactam like impurities that have the potential to cause
anaphylaxis and which currently do not have any threshold of concern [23].
It is worth highlighting that
(a) These impurities are extremely rare and do not reflect the typical structure of impurities generated by medicinal research [26,27] and
(b) That these structural motifs (if present) would be highlighted and addressed as part of the ICH M7 risk assessment [5] , as "the findings from any mutagenic risk assessment are also reviewed by toxicology experts"
Conclusion
Safety based impurity limits are a core consideration
of all of the existing ICH Q3 guidance documents. However, there has
been an evolution in the approach toward impurities since the
publication of the initial guidance [2,6]. Whereas, ICH Q3A [2] and Q3B [6]
provide general guidance on impurities in drug substances and drug
products, respectively and mainly focus on absolute levels of
impurities, i.e. percentage based limits; later guidance focused on
individual impurity classes; i.e. residual solvents [11], residual elemental impurities [14] and mutagenic impurities [5]
and had a greater focus on daily exposure limits. In the latter cases,
this led to the introduction of various impurity specific limits, such
as PDEs, Als, TTCs and staged TTCs, all aimed at defining a virtually
safe dose (VSD). This in turn led to the introduction of LTL limits for
mutagenic impurities.
LTLs are based on an application of Haber's law which
states that concentration and exposure times are both critical for
assessing likely safety risk to patients. Surprisingly, LTLs have not
been applied to the other specific classes of impurities or indeed
general impurities. In order to address this deficiency, Harvey et al. [23] have assessed the underpinning data behind the current "1mg or 1%, whichever is lower" limit in ICH Q3A [2] /Q3B [6],
and they found this to be based on robust science and they proposed an
ancillary LTL for general impurities of 5 mg or 0.7%, whichever is
lower, for clinical studies with durations of less than 6 months.
Logically, the toxicity of the parent drug substance
also affects how we deal with impurities, even very toxic impurities.
Thus, there is limited, if any, additional impact on patient safety for
impurities where the parent drug substance is mutagenic, carcinogenic or
cytotoxic. Accordingly it was recognised that for oncology products,
impurity levels (even mutagenic impurities) could be controlled at
higher levels. This "higher level" wasn’t defined but is based on an
overview of the disease being treated, including patient prognosis, and
the nature of the drug itself. Additionally, from a risk based
perspective and an understanding of Haber's law, an overt focus on
impurity control makes little sense if the life expectancy of the
affected patient is short, i.e. less than 2 years [20].
Interestingly, there has been little regulatory appetite for widening
this entirely pragmatic approach to impurity control to other
therapeutic areas, where life expectancy is equally short, i.e. rare
diseases [22].
For more Open Access Journals in Juniper
Publishers
For more articles in Global Journal
of Pharmacy & Pharmaceutical Sciences please
Comments
Post a Comment